Comprehensive PEF System Analysis and Microalgae Applications
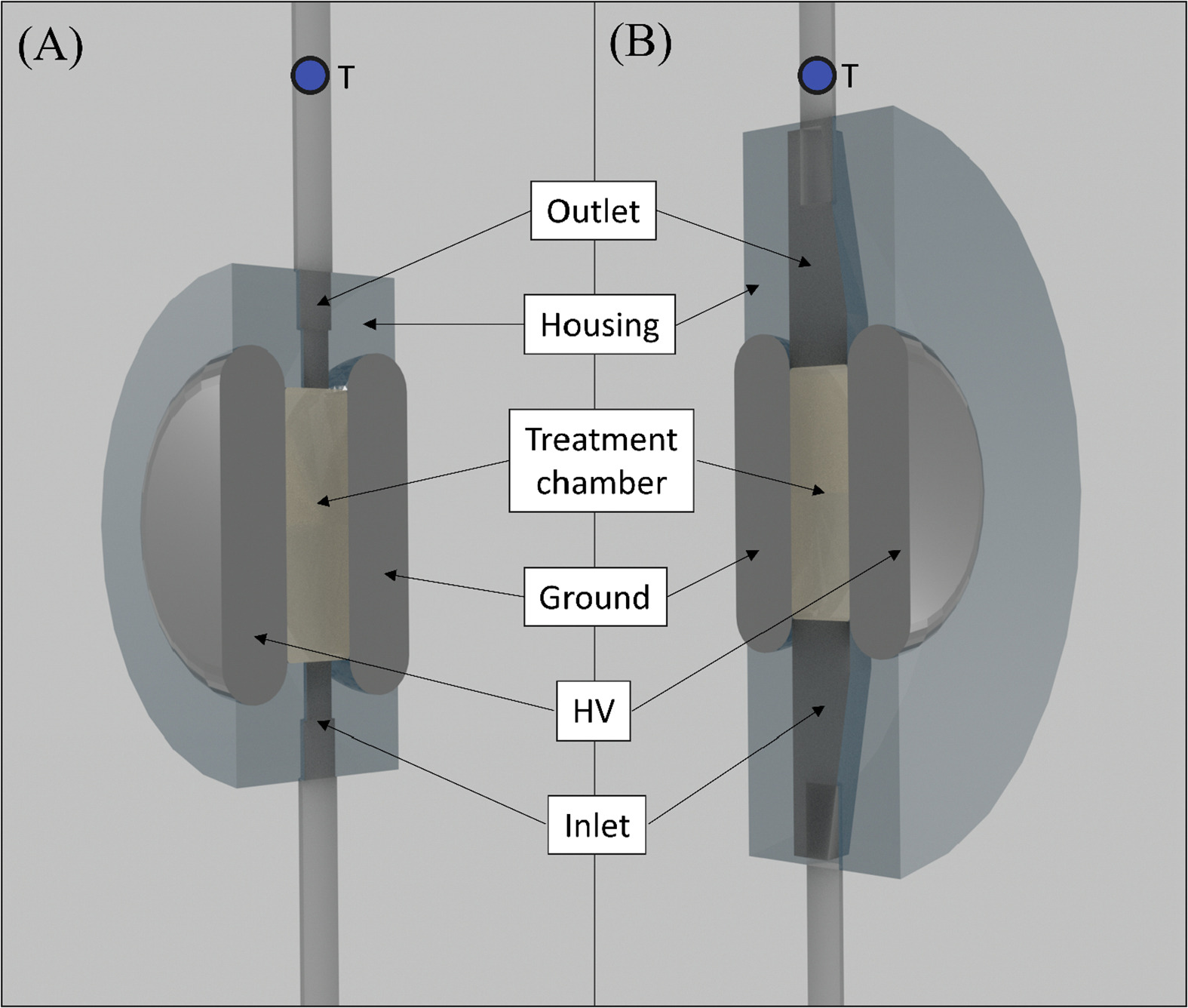
Pulsed electric field (PEF) is an emerging nonthermal technique with promising applications in microalgae biorefinery concepts. In this work, the flow field in continuous PEF processing and its influencing factors were analyzed and energy input distributions in PEF treatment chambers were investigated. The results were obtained using an interdisciplinary approach that combined multiphysics simulations with ultrasonic Doppler velocity profiling (UVP) and rheological measurements of algae suspensions as a case study for applications in the biobased industry. UVP enabled non-invasive validation of multiphysics simulations. A. platensis suspensions follow a non-Newtonian, shear-thinning behavior, and measurement data could be fitted with rheological functions, which were used as an input for fluid dynamics simulations. Within the present work, a comprehensive system characterization was achieved that will facilitate research in the field of PEF processing.
In the 2nd figure, a processing window applying three treatments of 100 ns, 5 Hz, and 10 kV cm−1 to industrially relevant phototrophic Chlorella vulgaris in the early exponential growth phase significantly increased biomass yields by up to 17.53 ± 10.46% (p = 3.18 × 10−5). Treatments had limited effects on the carbon and pigment contents, but the protein content was decreased. The longest possible pulse width (100 ns) resulted in the highest biomass yield indicating underlying working mechanisms of enhanced cell proliferation based on intracellular and plasma membrane-related effects. The applicability to eukaryotes and prokaryotes, such as C. vulgaris and cyanobacteria highlights the possible impacts of nsPEF across multiple domains of the biobased industry relying on single-cell-based value-chains.
In the 3rd figure, an overview of these effects is shown. Conventional PEF and the emerging area of nanosecond PEF (nsPEF) have been shown to induce various biological effects, with nsPEF inducing pronounced intracellular effects, which could provide solutions for currently faced challenges. Based on the flexibility and continuous operation of PEF and nsPEF processing, the technology can be integrated into many existing cultivation systems; its modularity provides an approach for inducing specific effects. Depending on the treatment conditions, selective inactivation, continuous extraction without impeding cell viability, as well as the stimulation of cell growth and/or cellular compound stimulation are potential applications in the bio-based industry. However, continuous treatment currently involves heterogeneous energy inputs. Increasing the homogeneity of PEF and nsPEF processing by considering the flow and electric field heterogeneity may allow for more targeted effects on biological cells, further increasing the potential of the technology for bio-based applications. We provide an overview of existing and potential applications of PEF and nsPEF and suggest that theoretical and practical analyses of flow and electric field heterogeneity may provide a basis for obtaining more targeted effects on biological cells and for further increasing the bio-based applications of the technology, which thereby could become a key technology for circular economy approaches in the future.
In the 4th figure, we show treatment responses of biological cells exposed to an electric field during nsPEF processing. Main effects reported relate to nanopore formation on membranous structures and an activation of voltage-gated and Ca2+-influx channels (organelle and plasma membrane), which can result in a mass transfer of molecules across membranes for instance causing transient, cytosolic Ca2+ hubs. The formation of reactive oxygen species (ROS), such as superoxide (·O2−) or hydrogen peroxide (H2O2) and nitrogen species (RNS), such as nitric oxide (NO·) was also reported. ROS/RNS formation occurs instantaneously or through the involvement of NADPH oxidase Rboh. ROS, RNS, and Ca2+ affect intracellular signaling pathways including those associated with auxin, mitogen-activated protein kinases (MAPK), such as the c-Jun N-terminal kinase (JNK), and nitric oxide (NO) signaling. NsPEF treatments caused Phosphatidylinositol-4,5-bisphosphate (PIP2) cleavage into Diacylglyerol (DAG) and Inositol trisphosphate (IP3), resulting in the release of Ca2+ from intracellular storage compartments and finally the induction of protein kinase-c (PKC)-dependent signaling pathways. Dissolution of the microfilament (actin) meshwork organization is also shown.
Last figure 5: Schematic of longitudinal section of (A) cylindrical inlet/outlet and (B) diffuser inlet/outlet setup leading into and out of the μsPEF treatment chamber (V = 3.5 mL, electrode gap size = 1 cm). Ground and high voltage (HV) refer to the respective stainless-steel electrodes. The T-labelled circle indicates where the temperature measurement occurred. Continuous μsPEF treatments were applied by connecting a RUP6-15CL pulse generator (GBS-Elektronik GmbH, Radeberg, Germany) to two different rectangular treatment chambers with a treatment volume (V) of 3.5 mL and electrode gap size (L) of 1 cm: Fig. 5 (A) cylindrical inlet/outlet (polycarbonate-based, manufactured at ETH Zurich, Switzerland) and (B) diffuser inlet/outlet (polypropylene-based, manufactured at HES-SO VS, Switzerland). The electrodes were made from stainless steel, as described elsewhere (Buchmann, Bloch, & Mathys, 2018; Goettel, Eing, Gusbeth, Straessner, & Frey, 2013).
Selected publications
Buchmann, L., Bloch, R. & Mathys A. 2018. Comprehensive pulsed electric field (PEF) system analysis for microalgae processing. Bioresource Technology 265, 268-274. external page DOI
Axelrod R., Baumgartner J., Tanner E., Beyrer M. & Mathys A. 2021. Effects of microsecond pulsed electric field (μsPEF) and modular micro reaction system (MMRS) treatments on whey protein aggregation. International Dairy Journal, vol. 123, pp. 105170, external page DOI
Haberkorn, I., Buchmann, L., Häusermann, I. & Mathys, A. 2021. Nanosecond pulsed electric field processing of microalgae based biorefineries governs growth promotion or selective inactivation based on underlying microbial ecosystems. Bioresource Technology, 319, 124173. external page DOI
Haberkorn, I., Siegenthaler, L., Buchmann, L., Neutsch, L. & Mathys, A. 2021. Enhancing single-cell bioconversion efficiency by harnessing nanosecond pulsed electric field processing. Biotechnology Advances, 319, 124173. external page DOI
Buchmann, L., Frey, W., Gusbeth, C., Ravaynia, P.S. & Mathys A. 2019. Effect of nanosecond pulsed electric field treatment on cell proliferation of microalgae. Bioresource Technology,271, 402-408. external page DOI
Haberkorn, I., Buchmann, L., Hiestand, M. & Mathys, A. 2019. Continuous nanosecond pulsed electric field treatments foster the upstream performance of Chlorella vulgaris-based biorefinery concepts. Bioresource Technology, 122029. external page DOI
Buchmann, L., & Mathys, A. 2019. Perspective on Pulsed Electric Field Treatment in the Bio-based Industry. Frontiers in Bioengineering and Biotechnology, vol. 7, pp. 265. external page DOI
Buchmann, L., Brändle, I., Haberkorn, I., Hiestand, M., & Mathys, A. 2019. Pulsed electric field based cyclic protein extraction of microalgae towards closed-loop biorefinery concepts. Bioresource Technology, vol. 291, pp. 121870, external page DOI
Buchmann, L., Bertsch, P., Böcker, L., Krähenmann, U., Fischer, P. & Mathys, A. 2019. Adsorption kinetics and foaming properties of soluble microalgae fractions at the air/water interface. Food Hydrocolloids, 105182. external page DOI
Buchmann, L., Böcker, L., Frey, W., Haberkorn, I., Nyffeler, M. & Mathys, A. 2018. Energy input assessment for nanosecond pulsed electric field processing and its application in a case study with Chlorella vulgaris. Innovative Food Science and Emerging Technologies 47, 445-453. external page DOI